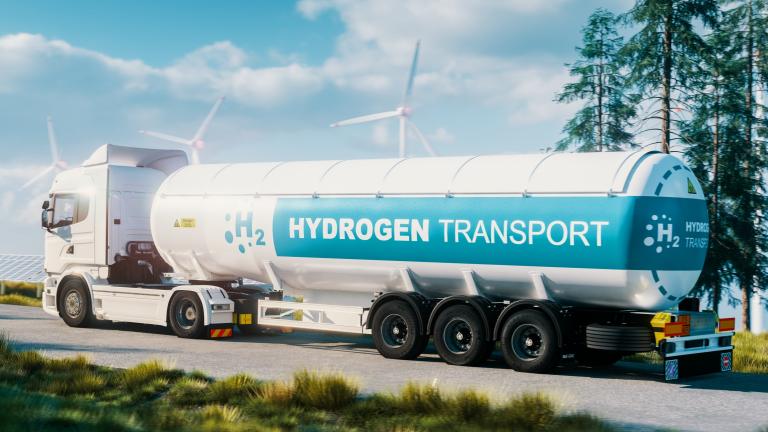
Over the past decades, the need for carbon-free energy has driven increasing interest in hydrogen as an environmentally clean fuel. But shifting the economy away from fossil fuels to clean-burning hydrogen will require significant adjustments in current supply chains. To facilitate this transition, an MIT-led team of researchers has developed a new hydrogen supply chain planning model.
“We propose flexible scheduling for trucks and pipelines, allowing them to serve as both storage and transmission,” says Guannan He, a postdoc at the MIT Energy Initiative (MITEI) and lead author of a recent paper published by IEEE Transactions on Sustainable Energy. “This is very important to green hydrogen produced from intermittent renewables, because this can provide extra flexibility to meet variability in supply and demand.”
Hydrogen has been widely recognized as a promising path to decarbonizing many sectors of the economy because it packs in more energy by weight than even gasoline or natural gas, yet generates zero emissions when used as an energy source. Producing hydrogen, however, can generate significant emissions. According to the U.S. Office of Energy Efficiency and Renewable Energy, 95 percent of the hydrogen produced today is generated through steam methane reforming (SMR), an energy-intensive process in which methane reacts with water to produce hydrogen and carbon monoxide. A secondary part of this process adds steam to the cooled gas to convert carbon monoxide to carbon dioxide (CO2) and produce more hydrogen.
Ultimately, hydrogen production today accounts for about 4 percent of CO2 emissions globally, says He, and that number will rise significantly if hydrogen becomes popular as a fuel for electric vehicles and such industrial processes as steel refining and ammonia production. Realizing the vision of creating an entirely decarbonized hydrogen economy therefore depends on using renewable energy to produce hydrogen, a task often accomplished through electrolysis, a process that extracts hydrogen from water electrochemically.
However, using renewable energy requires storage to move energy from times and places with peak generation to those with peak demand. And, storage is expensive.
The researchers expanded their thinking about storage to address this key concern: They used trucks in their model both as a means of fuel transmission and of storage — since hydrogen can be readily stored in idled trucks. This tactic reduces costs in the hydrogen supply chain by about 9 percent by bringing down the need for other storage solutions, says He. “We found it very important to use the trucks in this way,” says He. “It can reduce the cost of the system and encourage renewable-based hydrogen production, instead of gas-based production.”
Developing the model
Previous studies have attempted to assess the potential benefit of hydrogen storage in power systems, but they have not considered infrastructure investment needs from the perspective of a whole hydrogen supply chain, He says. And such work is critical to enabling a hydrogen economy.
For the new model, the research team — He; MITEI research scientists Emre Gençer and Dharik Mallapragada; Abhishek Bose, an MIT master’s student in technology and policy; and Clara F. Heuberger, a researcher at Shell Global Solutions International B.V. — adopted the perspective of a central planner interested in minimizing system costs and maximizing societal benefit. The researchers looked at costs associated with the four main steps in the hydrogen supply chain: production, storage, compression, and transmission. “Unless we take a holistic approach to analyzing the entire supply chain, it is hard to determine the prospects for hydrogen. This work fills that gap in the literature,” Gençer says.
To ensure their model was as comprehensive as possible, the researchers included a wide range of hydrogen-related technologies, including SMR with and without carbon capture and storage, hydrogen transport as a gas or liquid, and transmission via pipeline and trucks. “We have developed a scalable modeling and decision-making tool for a hydrogen supply chain that fully captures the flexibility of various resources as well as components,” Gençer says.
While considering all options, in the end the researchers found that pipelines were a less flexible option than trucks for transmission (although retrofitting gas pipelines could make hydrogen pipelines cost-effective for some uses), and trucking hydrogen gas was less expensive than trucking hydrogen in liquid form, since liquefaction has much higher energy consumption and capital costs than gas compression.
They then proposed a flexible scheduling and routing model for hydrogen trucks that would enable the vehicles to be used as both transmission and storage, as needed. Computationally, this was a particularly challenging step, according to He. “This is a very complex optimization model,” he says. “We propose some techniques to reduce the complexity of the model.”
The team chose to use judicious approximations for the number of trucks in the system and the needed commitment of SMR units, applying clustering and integer relaxation techniques. This enabled them to greatly improve the computational performance of their program without significantly impacting results in terms of cost and investment outcomes.
Case study of Northeast
Once the model was built, the researchers tested it by exploring the future hydrogen infrastructure needs of the U.S. Northeast under various carbon policy and hydrogen demand scenarios. Using 20 representative weeks from seven years of data, they simulated annual operations and determined the optimal mix of hydrogen infrastructure types given different carbon prices and the capital costs of electrolyzers.
“We showed that steam methane reforming of natural gas with carbon capture will constitute a significant fraction of hydrogen production and production capacity even under very high carbon price scenarios,” Gençer says.
However, He says the results also suggest there is real synergy between the use of electrolysis for hydrogen generation and the use of compressed-gas trucks for transmission and storage. This finding is important, he explains, because “once we invest in these assets, we cannot easily switch to others.”
He adds that trucks are a significantly more flexible investment than stationary infrastructure, such as pipes and transmission lines; trucks can easily be rerouted to serve new energy-generation facilities and new areas of demand, or even be left sitting to provide storage until more transmission capacity is needed. By comparison, building new electricity transmission lines or pipelines takes time — and they cannot be quickly adapted to changing needs.
“You have more renewables integrated into the system every day. People are installing rooftop solar panels, so you need more assets to transmit energy to other parts of the system,” He says, explaining that a flexible supply chain can make the most of renewable generation. “A transmission line can take 10 years to build, during which time those renewables cannot be used as well. Using smaller-scale, distributed, portable storage or mobile storage can solve this problem in a timely manner.”
Indeed, He and other colleagues recently conducted related research into the potential application of utility-scale portable energy storage in California. In a paper published in Joule in February, they showed that mobilizing energy storage can significantly increase revenues from storage in many regions and improve renewable energy integration. “It’s more flexible” than such stationary solutions as additional grid capacity, He says. “When you don’t need mobile storage anymore, you can convert it into stationary storage.”
Now that He and his colleagues have created their hydrogen supply chain planning model, the next step, according to He, is to provide planners with broad access to the tool. “We are developing open-source code so people can use it to develop optimal assets for different sectors,” He says. “We are trying to make the model better.”
This research was supported by Shell New Energies Research and Technology and the MIT Energy Initiative Low-Carbon Energy Centers for Electric Power Systems and Carbon Capture, Utilization, and Storage. The research reported in Joule was supported by the National Natural and Science Foundation of China and a grant from the U.S. Department of Energy.