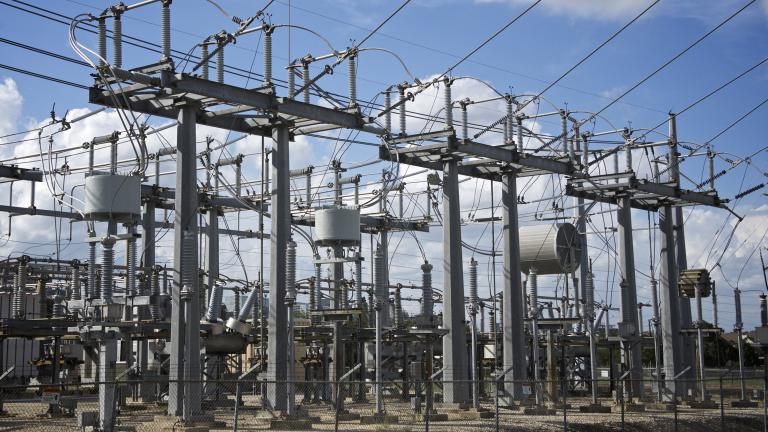
In almost all US states, electric utilities have been recovering distribution costs, along with electricity supply costs, from residential and small commercial customers based on monthly electricity consumption (in kWh), regardless of the timing of that consumption. We refer to these as flat volumetric tariffs. As there is broad consensus that flat volumetric network tariffs are not cost-reflective (Pérez-Arriaga et al., 2017) and discourage electrification (Schittekatte et al., 2023), regulatory commissions across the US have taken a range of approaches to designing alternative tariffs. Recently, an increasing number of states have enacted policies to make time-of-use (TOU) rates the default option (Kavulla, 2023). In all these states there is no retail competition, so the distribution utility is also the energy supplier, and the TOU rate bundles supply, distribution, and transmission costs.
This evolution is a step in the right direction; TOU rates, when well designed, can provide end users with relatively good incentives to shift load from periods with high to low marginal supply costs at the bulk power system level (Schittekatte et al., 2024). However, when local concentrations of EVs (or other shiftable load) rise and wholesale price patterns are poorly aligned with demands on distribution networks, TOU rates may increase network congestion. A per-kWh charge alone, even a time-differentiated one, provides no disincentive for consumers to limit their maximum instantaneous kW consumption. As consumers defer EV charging to off-peak hours, TOU rates may result in local demand spikes at the onset of off-peak periods, potentially leading to steeply rising costs for distribution network upgrades.
Figure 1 illustrates this issue, showing average hourly electricity demand for a simulated neighborhood in which 30% of households have EVs and seek to minimize charging costs. The left panel shows the aggregated load under flat volumetric tariffs, which are most common today. The right panel shows the same under a bundled TOU rate, with the off-peak period beginning at 9:00 PM. Under the status quo, the local peak increases due to EV adoption; vehicles begin charging immediately upon arriving home but heterogeneity in arrival time leads to some spreading of charging over the evening hours. Under the bundled TOU rate, all vehicles that arrive home prior to 9:00 PM delay charging to start until that hour (when the electricity cost is cheaper), producing a large demand increase at the onset of the off-peak period. We find under bundled TOU pricing, this correlated EV charging becomes a serious issue even at low EV adoption levels, with newly created local demand peaks appearing as early as 15% adoption. This is even more concerning when considering that EV adoption is highly clustered spatially and will not proceed uniformly across a distribution utility’s service territory.
Figure 1. Average hourly weekday electricity demand aggregated across 400 households with 30% EV adoption under a flat volumetric tariff (left) and bundled TOU tariff (right)
Under the flat volumetric tariff, EVs start charging immediately upon arrival. Under the bundled TOU tariff, EVs delay charging until the start of the off-peak period at 9:00 PM.
In this paper, we study how to complement TOU supply charges with separate distribution network tariffs to deal with the problem of bunched EV charging. To perform the analysis, we conduct a realistic case study using simulated residential load and driving profiles at increasing levels of EV adoption, calibrated for 400 households in Massachusetts. We study three types of network tariffs – fixed (per-connection), volumetric (per-kWh), and capacity (per-kW) – and analyze the results of households individually minimizing their electricity costs. All network tariffs are paired with a 2-part TOU supply tariff. We introduce two types of per-kW charges that have been in place for residential consumers in several European countries for many years. First, demand charges for which the maximum (ex-post) measured peak demand during a predefined time period determines the network charge. Second, subscription charges for which end users contract ex-ante for a maximum per-kW level they want to have access to at all times during a predefined time period. We run two scenarios (low and high) representing a range of possible network upgrade costs and consider three key metrics to compare the performance of the different network tariffs: annual local peak demand (which drives network investments), levelized cost of EV charging, and changes in network charges for non-EV households. The paper includes a review of network tariffs prevalent in Europe and both default TOU and EV-specific tariffs active in the US. We also review the academic literature on network tariff design in the face of increasing consumer adoption of distributed energy resources.
Figure 2 shows the simulated neighborhood’s annual peak demand under all networks tariff tested at 5% EV adoption increments. The right panel zooms in on 0 – 30% EV adoption to highlight the divergence in tariff performance even at low adoption levels. The fixed, 1-part per-kWh and 2-part TOU per-kWh tariffs produce the same annual peak result because the network tariff does not impact the shifting incentives from the common TOU supply charge (the 2-part TOU per-kWh network tariff has the same on/off-peak structure as the 2-part TOU supply charge).
Figure 2. Annual peak demand at 5% EV adoption increments for seven network tariffs tested (left), with 0 – 30% adoption magnified (right)
As early as 15% adoption, fixed and per-kWh tariffs diverge sharply from capacity-based tariffs, which incentivize households to spread out charging demand.
A capacity tariff outperforms fixed and volumetric tariffs in terms of annual peak because it provides an incentive to charge at a level lower than the charging equipment’s technical potential, illustrated in Figure 3. The left panel shows the demand profile of an individual household during one 24-hour period under a bundled TOU tariff compared to under a subscription network tariff where the household manages EV charging to stay below its contracted capacity of 3.0 kW. Even without centralized control, when all households behave like this, acting independently to manage their own demand, the result is a lower aggregate peak compared to a bundled TOU rate, shown in the right panel.
Figure 3. The impact of a subscription tariff.
Example of an individual household reacting to a subscription tariff versus a bundled TOU tariff (left) and average hourly weekday electricity demand aggregated across all 400 households for the subscription tariff versus bunded TOU tariff at 30% EV adoption (right). Note that the scales for the vertical demand axes are not equal. Under a subscription tariff, households still delay charging until the start energy off-peak period, but the peak is less pronounced because households have an incentive to manage charging to stay below their contracted capacity.
Table 1 shows the results of the three metrics for the different network tariffs under 50% EV adoption. Per-kWh network charges lead to high EV charging costs and lack a price signal to limit aggregated demand peaks (hence increasing the total network costs to be recovered). Fixed network charges foster electrification by lowering the cost of EV charging, yet they shift costs from EV owners to others and again lack a mechanism to mitigate peak demand. Capacity-based tariffs (demand and subscription charges) offer a compromise, providing a significant reduction in levelized charging cost compared to per-kWh tariffs while increasing network costs for non-EV owners by only a modest amount compared to a situation without EV adoption. These network tariffs, complementing TOU supply charges, are a pragmatic approach to better control the impacts of rising EV penetration on network costs; incentivizing electrification (a priority for many US states) need not be pursued at the expense of broader affordability goals.
Table 1. Key metrics for each network tariff at 50% EV adoption under low and high network upgrade cost scenarios (i.e., LRMC)
There is a tradeoff among assessment criteria; the fixed tariff performs best in levelized charging cost but shifts costs to non-EV owners. Per-kWh network tariffs protect non-EV owners but increase charging costs. Capacity-based tariffs offer a compromise.
While a 3-part seasonal demand charge achieves the lowest annual peak (and associated distribution network costs) in our case study, such a rate design is difficult to explain to customers and does not provide protections against bill shocks. In contrast, a subscription charge performs reasonably well for all considered assessment criteria. As Public Utility Commissions attempt to balance stakeholder interests in promoting electrification, a tariff design that does not create big winners or losers may be the most palatable. And whereas some tariff designs rely on perfectly rational consumer behavior to achieve their desired impact, we show in the paper how a subscription charge’s performance on all criteria actually improves when a small portion of customers ignore price signals. Further we argue that subscription tariffs have several implementation advantages.
First, if a customer must subscribe in advance and is required to resubscribe from time to time – e.g., with estimated savings and a default option to continue at the same level – it forces them to think about how they can minimize costs. When the demand charge just gets buried in the tariff, they may not focus their attention on minimizing total cost. In contrast, under a subscription charge smart meters are typically programmed such that the meter is temporarily disconnected if instantaneous demand exceeds the subscribed level. This immediate feedback will help coach customers to not turn on multiple high-power devices simultaneously or to purchase devices that make it possible to program which appliances get turned off first (Mou et al., 2017). Second, a subscription offers more bill certainty, which is important for customers on tight monthly budgets. Even without perfect foresight, customers can better predict their costs using their subscribed value compared with a demand charge charged after the fact. Third, a subscription structure is similar to popular phone and internet plans, whereby customers pay for a maximum level of service that cannot be exceeded without incurring penalties. A familiarity with these types of plans will help explain the logic of subscription charges and ease the transition to new network tariffs. Fourth and last, customers signing up for certain levels of maximum demand to which they want to have access better aligns the horizon of consumer decisions with the horizon of network planning, i.e., subscription plans can help utilities to plan future networks.
In summary, our results urge utilities and their regulators to consider the importance of separating network charges from TOU supply charges and implementing a subscription network tariff. This recommendation is not exclusive to states with vertically integrated utilities but can equally be applied to states with unbundled tariffs (e.g. the three California IOUs) or retail competition. In the latter cases, while the separation between energy and network costs is inherent to the regulatory model, currently often flat or TOU per-kWh tariffs are in place to recoup distribution costs. A well-designed subscription tariff has the potential to 1) mitigate the need for local capacity upgrades, especially at early adoption levels, 2) provide low levelized charging costs for EV owners, a key motivator for EV adoption, and 3) reduce the cost burden on non-EV households.
Further Reading:
CEEPR WP 2024-02
Citations:
Kavulla, Travis (2023) “Why Is the Smart Grid So Dumb? Missing Incentives in Regulatory Policy for an Active Demand Side in the Electricity Sector.” Energy Systems Integration Group. https://www.esig.energy/wp-content/uploads/2023/01/Why-Is-the-Smart-Grid-So-Dumb-Missing-Incentives-in-Regulatory-Policy-for-an-Active-Demand-Side-in-the-Electricity-Sector.pdf
Mou, Yuting, Anthony Papavasiliou, and Philippe Chevalier (2017) “Application of Priority Service Pricing for Mobilizing Residential Demand Response in Belgium.” In 2017 14th International Conference on the European Energy Market (EEM), 1–5. IEEE. https://ieeexplore.ieee.org/abstract/document/7981860/?casa_token=7rjp8qP5vBcAAAAA:8WL5sYVaTv-OA5O0o4plLLJrHd7GNhAuskZQYmbjN0QmCrIXkVhzUzMj1riz5fqhL9j5oaro0es.
Pérez-Arriaga, Ignacio J., Jesse D. Jenkins, and Carlos Batlle (2017) “A Regulatory Framework for an Evolving Electricity Sector: Highlights of the MIT Utility of the Future Study.” Economics of Energy & Environmental Policy 6 (1): 71–92.
Schittekatte, Tim, Dharik Mallapragada, Paul L. Joskow, and Richard Schmalensee (2023) “Reforming Retail Electricity Rates to Facilitate Economy-Wide Decarbonization.” Joule. https://www.cell.com/joule/pdf/S2542-4351(23)00124-1.pdf.
——— (2024) “Electricity Retail Rate Design in a Decarbonizing Economy: An Analysis of Time-of-Use and Critical Peak Pricing.” The Energy Journal 45 (3). https://doi.org/10.5547/01956574.45.3.tsch.